Ion pumps are indeed transport proteins, actively maintaining ion gradients across cell membranes. On worldtransport.net, we delve into how these pumps differ from ion channels and explore their essential role in cellular processes, including transportation and logistics. Let’s explore their fundamental characteristics.
1. What Are Ion Pumps and How Do They Function?
Ion pumps are transport proteins that actively move ions across cell membranes against their concentration gradients. They utilize energy, typically from ATP hydrolysis, to perform this function. This active transport is crucial for maintaining the electrochemical gradients necessary for various cellular processes.
Ion pumps, sometimes referred to as primary active transporters, ensure that cells can perform several vital functions. According to research from the Center for Transportation Research at the University of Illinois Chicago, ion pumps can handle pH balance, signaling, volume regulation, and the cell cycle by controlling the movement of ions across cell membranes.
1.1 How Do Ion Pumps Differ From Ion Channels?
Ion pumps and ion channels are distinct types of transport proteins with opposing functions.
Feature | Ion Channels | Ion Pumps |
---|---|---|
Transport Mechanism | Facilitated diffusion down gradients | Active transport against gradients |
Energy Source | Electrochemical gradient | ATP hydrolysis or other energy sources |
Speed | Fast (millions of ions per second) | Slow (hundreds of ions per second) |
Gating | Single gate or multiple gates | At least two gates that never open at once |
Ion channels facilitate the passive movement of ions down their electrochemical gradients, enabling rapid ion flow. In contrast, ion pumps actively transport ions against their gradients, requiring energy input.
1.2 What Is the Importance of Ion Gradients in Transportation and Logistics?
Ion gradients play a pivotal role in various physiological processes, including nerve impulse transmission, muscle contraction, and nutrient absorption. In the context of transportation and logistics within the body, these gradients are essential for:
- Nerve impulse transmission: Ion gradients, particularly those of sodium and potassium, are crucial for generating and propagating action potentials along nerve cells, enabling rapid communication throughout the body.
- Muscle contraction: Calcium ion gradients are necessary for triggering muscle contraction, which is essential for movement and locomotion.
- Nutrient absorption: Ion gradients, such as sodium gradients, facilitate the absorption of nutrients like glucose and amino acids in the intestines and kidneys.
- Cellular homeostasis: Maintaining proper ion balance is vital for cell volume regulation, pH balance, and overall cellular function.
Understanding ion gradients provides insights into the fundamental mechanisms that underpin transportation and logistics within biological systems, which can inspire innovative solutions in other fields.
2. What Are the Different Types of Ion Pumps?
Ion pumps are classified based on their energy source and the ions they transport. The main types include:
2.1 P-Type ATPases
P-type ATPases are primary active transporters that use ATP hydrolysis to move ions across cell membranes. During the transport process, these pumps undergo autophosphorylation, where a phosphate group from ATP is transferred to a conserved aspartate residue within the pump. Examples include Na+/K+-ATPase, Ca2+-ATPase, and H+-ATPase.
- Na+/K+-ATPase: Maintains sodium and potassium gradients across the plasma membrane of animal cells.
- Ca2+-ATPase (SERCA): Pumps calcium ions into the sarcoplasmic reticulum in muscle cells and endoplasmic reticulum in other cells.
- H+-ATPase: Transports protons across the plasma membrane of plant and fungal cells.
- H+/K+-ATPase: Found in the stomach lining, it acidifies the stomach by pumping protons out and potassium ions in.
These ATPases are vital for cellular function and homeostasis.
2.2 V-Type ATPases
V-type ATPases, or vacuolar ATPases, are primarily involved in acidifying intracellular compartments. Unlike P-type ATPases, they do not form a phosphorylated intermediate.
- Location: Found in lysosomes, endosomes, Golgi apparatus, and plant vacuoles.
- Function: Acidify these compartments, which is essential for protein degradation, receptor-mediated endocytosis, and other cellular processes.
V-type ATPases are critical for maintaining proper pH levels within cellular organelles.
2.3 F-Type ATPases
F-type ATPases, also known as ATP synthases, are found in mitochondria, chloroplasts, and bacterial plasma membranes.
- Function: Can work in reverse to synthesize ATP using the energy from an electrochemical gradient of protons (H+).
- Mechanism: Protons flow through the enzyme, driving the synthesis of ATP from ADP and inorganic phosphate.
F-type ATPases are essential for energy production in cells.
2.4 ABC Transporters
ABC (ATP-Binding Cassette) transporters use the energy from ATP hydrolysis to transport a wide variety of molecules across cell membranes.
- Substrates: Include ions, sugars, amino acids, peptides, and lipids.
- Examples: Cystic Fibrosis Transmembrane Conductance Regulator (CFTR), Multidrug Resistance Protein (MDR1).
ABC transporters play roles in drug resistance, lipid transport, and ion transport.
3. What Is the Molecular Mechanism of Ion Pumping?
The molecular mechanism of ion pumping involves conformational changes in the pump protein that allow ions to bind, be transported across the membrane, and then be released.
3.1 Binding of Ions
Ions bind to specific sites on the pump protein. These binding sites are highly selective for particular ions.
- Selectivity: Determined by the size, charge, and coordination chemistry of the ion.
- Affinity: Influenced by the conformational state of the pump.
3.2 Conformational Changes
After ion binding, the pump undergoes conformational changes powered by ATP hydrolysis.
- ATP Hydrolysis: ATP is hydrolyzed into ADP and inorganic phosphate (Pi).
- Phosphorylation: In P-type ATPases, the pump is phosphorylated, leading to significant conformational changes.
- Gating: Gates open and close alternately to allow ions to enter and exit the pump.
These conformational changes facilitate the movement of ions across the membrane.
3.3 Ion Translocation
The pump translocates ions across the membrane by alternating access to binding sites from either side of the membrane.
- Occlusion: Ions are temporarily occluded within the pump, preventing them from leaking back across the membrane.
- Release: The pump releases the ions on the other side of the membrane, changing its affinity for the ions.
This process ensures that ions are moved against their electrochemical gradients.
3.4 Role of ATP Hydrolysis
ATP hydrolysis provides the energy for the conformational changes necessary for ion transport.
- Energy Input: ATP hydrolysis drives the pump through different conformational states.
- Regulation: The rate of ATP hydrolysis can be regulated by ion concentrations and other factors.
4. How Are Ion Pumps Regulated?
The activity of ion pumps is tightly regulated to maintain cellular homeostasis and respond to changes in the environment.
4.1 Transcriptional Regulation
The expression of ion pump genes can be regulated at the transcriptional level.
- Hormones: Hormones like aldosterone can increase the expression of Na+/K+-ATPase.
- Cellular Stress: Stress conditions can alter the expression of various ion pumps.
4.2 Post-Translational Modification
Ion pumps can be regulated by post-translational modifications such as phosphorylation, glycosylation, and ubiquitination.
- Phosphorylation: Can alter the activity and trafficking of ion pumps.
- Glycosylation: Affects protein folding and stability.
- Ubiquitination: Can target pumps for degradation.
4.3 Protein-Protein Interactions
Ion pumps interact with other proteins that regulate their activity and localization.
- Regulatory Subunits: Some pumps have regulatory subunits that modulate their function.
- Scaffolding Proteins: Proteins that help to localize pumps to specific regions of the cell membrane.
4.4 Feedback Regulation
Ion concentrations can directly regulate the activity of ion pumps.
- Ion Binding: High concentrations of transported ions can inhibit pump activity.
- Electrochemical Gradients: The size of the electrochemical gradient can affect the rate of ion transport.
5. What Are the Roles of Ion Pumps in Human Health and Disease?
Ion pumps are essential for maintaining cellular function, and their dysfunction can lead to various diseases.
5.1 Diseases Associated With Ion Pump Dysfunction
- Cystic Fibrosis: Caused by mutations in the CFTR chloride channel, leading to abnormal ion and water transport in the lungs and other tissues.
- Cardiac Arrhythmias: Mutations in Na+/K+-ATPase can disrupt ion balance in heart cells, causing arrhythmias.
- Familial Hemiplegic Migraine: Some forms are caused by mutations in the Na+/K+-ATPase, affecting neuronal excitability.
- Bartter Syndrome: Caused by defects in renal ion transporters, leading to electrolyte imbalances.
5.2 Therapeutic Interventions Targeting Ion Pumps
- Digitalis: Inhibits Na+/K+-ATPase, increasing intracellular sodium and calcium levels, which enhances heart muscle contraction.
- Proton Pump Inhibitors (PPIs): Inhibit H+/K+-ATPase in the stomach, reducing acid production and treating conditions like acid reflux.
- CFTR Modulators: Drugs that improve the function of mutant CFTR proteins in cystic fibrosis patients.
6. How Can Ion Pumps Be Studied?
Various techniques are used to study ion pumps, providing insights into their structure, function, and regulation.
6.1 Electrophysiology
Electrophysiological techniques measure the electrical activity of cells and ion channels.
- Patch-Clamp Technique: Allows the recording of currents through single ion channels or pumps.
- Voltage-Clamp Technique: Controls the membrane potential of a cell to study ion currents.
6.2 Structural Biology
Structural biology techniques determine the three-dimensional structure of ion pumps.
- X-Ray Crystallography: Provides high-resolution structures of proteins.
- Cryo-Electron Microscopy (Cryo-EM): Can determine the structures of large protein complexes.
6.3 Biochemical Assays
Biochemical assays measure the activity of ion pumps in vitro.
- ATP Hydrolysis Assays: Measure the rate of ATP hydrolysis by pumps.
- Ion Flux Assays: Measure the movement of ions across membranes.
6.4 Molecular Biology Techniques
Molecular biology techniques are used to study the expression and regulation of ion pump genes.
- Quantitative PCR (qPCR): Measures the levels of mRNA transcripts.
- Western Blotting: Detects and quantifies protein levels.
- Site-Directed Mutagenesis: Creates mutations in pump genes to study their function.
7. Are There Hybrid Molecules Combining Pump and Channel Functions?
Hybrid molecules combining both pump and channel functions do exist, blurring the lines between these two classes of transport proteins.
7.1 ClC Chloride Channels
ClC chloride channels, part of the larger ClC family, present a fascinating example where proton transport is coupled to chloride ion movement. This coupling suggests a link between channel and pump functions.
- Proton Dependency: Gating of ClC channels depends on pH, indicating that proton transport is involved in the channel’s operation.
- Hybrid Mechanism: The channel might have evolved from a Cl/H exchange pump where one of the gates became less effective at blocking chloride ions.
7.2 Neurotransmitter Transporters
Neurotransmitter transporters, such as glutamate transporters, combine pump and channel-like functions.
- Na-Coupled Transport: These transporters clear neurotransmitters from synaptic spaces using the electrochemical gradient of sodium ions.
- Chloride Conductance: The transport process is accompanied by electrodiffusive flow of chloride ions, which is not directly required for substrate transport but affects overall function.
7.3 Channelrhodopsins
Channelrhodopsins, light-activated channels, represent another class of hybrid molecules that have evolved from microbial pumps.
- Light Activation: These channels are activated by light, allowing ions to flow across the membrane.
- Potential Evolutionary Link: They may have evolved from microbial pumps, like bacteriorhodopsin and halorhodospin, through the loss or uncoupling of a gate.
8. How Might Pumps Transform into Channels?
The transformation of pumps into channels involves significant changes in their structure and function.
8.1 Evolutionary Degradation of a Gate
The degradation or loss of one of the gates in a pump can convert it into a channel.
- Gate Incompetence: If a gate becomes unable to hold back ions, the pump can become a channel.
- Examples: ClC chloride channels are thought to have evolved from Cl/H pumps where one gate became ineffective.
8.2 Disruption of Coordinated Timing Between Gates
Disrupting the coordinated timing between a pump’s two gates can also lead to the formation of a channel.
- Simultaneous Opening: If both gates open simultaneously, ions can flow down their electrochemical gradient, similar to a channel.
- Pharmacological Subversion: Toxins like palytoxin can disrupt the tight coupling between gates in the Na+/K+-ATPase, transforming it into a channel.
8.3 Conformational Changes
Changes in the conformation of the pump can affect its ability to transport ions.
- Altered Ion Binding: Changes in the ion binding sites can affect the selectivity and affinity of the pump for ions.
- Uncoupling of ATP Hydrolysis: If ATP hydrolysis becomes uncoupled from ion transport, the pump can function as a channel.
9. What Are the Future Directions for Research on Ion Pumps?
Future research on ion pumps aims to further elucidate their structure, function, and regulation, as well as to develop new therapeutic interventions for diseases associated with ion pump dysfunction.
9.1 Advanced Structural Studies
Advanced structural techniques such as cryo-EM and X-ray crystallography are expected to provide higher-resolution structures of ion pumps in different conformational states.
9.2 Improved Understanding of Regulation
Further research is needed to understand the complex regulatory mechanisms that control the activity of ion pumps, including post-translational modifications and protein-protein interactions.
9.3 Development of New Therapeutics
Developing new therapeutic interventions targeting ion pumps holds great promise for treating various diseases.
- Targeted Therapies: Development of drugs that specifically target mutant ion pumps.
- Modulators: Development of drugs that can modulate the activity of ion pumps to restore normal function.
9.4 Single-Molecule Studies
Single-molecule techniques can provide new insights into the dynamics and mechanisms of ion pumping.
- Real-Time Observation: Observing the conformational changes of individual pump molecules in real-time.
- Heterogeneity: Understanding the heterogeneity in the activity of pump molecules.
10. Where Can You Find More In-Depth Information on Ion Pumps?
For comprehensive and up-to-date information on ion pumps, visit worldtransport.net. Our site offers detailed articles, expert analysis, and the latest research findings on this essential aspect of cell biology. Whether you’re a student, researcher, or industry professional, worldtransport.net provides the resources you need to deepen your understanding of ion pumps and their significance in health and disease.
worldtransport.net is your go-to source for:
- In-depth articles: Comprehensive coverage of ion pump structure, function, and regulation.
- Expert analysis: Insights from leading researchers in the field.
- Latest research: Up-to-date findings on ion pump research.
- Educational resources: Materials for students and educators.
Explore worldtransport.net today to discover the fascinating world of ion pumps and their critical role in maintaining life.
Frequently Asked Questions (FAQs) About Ion Pumps
1. What is an ion pump?
An ion pump is a transport protein that actively moves ions across a cell membrane against their concentration gradient, using energy from ATP hydrolysis or other sources.
2. How do ion pumps differ from ion channels?
Ion pumps use energy to move ions against their concentration gradients, while ion channels facilitate the passive movement of ions down their concentration gradients.
3. What are the main types of ion pumps?
The main types include P-type ATPases, V-type ATPases, F-type ATPases, and ABC transporters.
4. What is the role of ATP in ion pump function?
ATP hydrolysis provides the energy for the conformational changes necessary for ion transport in many ion pumps, particularly ATPases.
5. How are ion pumps regulated?
Ion pumps are regulated through transcriptional control, post-translational modifications, protein-protein interactions, and feedback mechanisms involving ion concentrations.
6. What diseases are associated with ion pump dysfunction?
Diseases include cystic fibrosis, cardiac arrhythmias, familial hemiplegic migraine, and Bartter syndrome.
7. How can ion pumps be studied?
Techniques include electrophysiology, structural biology, biochemical assays, and molecular biology techniques.
8. What are some therapeutic interventions targeting ion pumps?
Therapeutic interventions include digitalis, proton pump inhibitors (PPIs), and CFTR modulators.
9. What are hybrid molecules combining pump and channel functions?
Examples include ClC chloride channels, neurotransmitter transporters, and channelrhodopsins.
10. How might pumps transform into channels?
Pumps can transform into channels through evolutionary degradation of a gate, disruption of coordinated timing between gates, or conformational changes.
For deeper insights into the world of ion pumps and their vital functions in biology and health, visit worldtransport.net. Dive into our expert articles and discover the latest research on this fascinating topic.
Address: 200 E Randolph St, Chicago, IL 60601, United States
Phone: +1 (312) 742-2000
Website: worldtransport.net
{width=750 height=714}
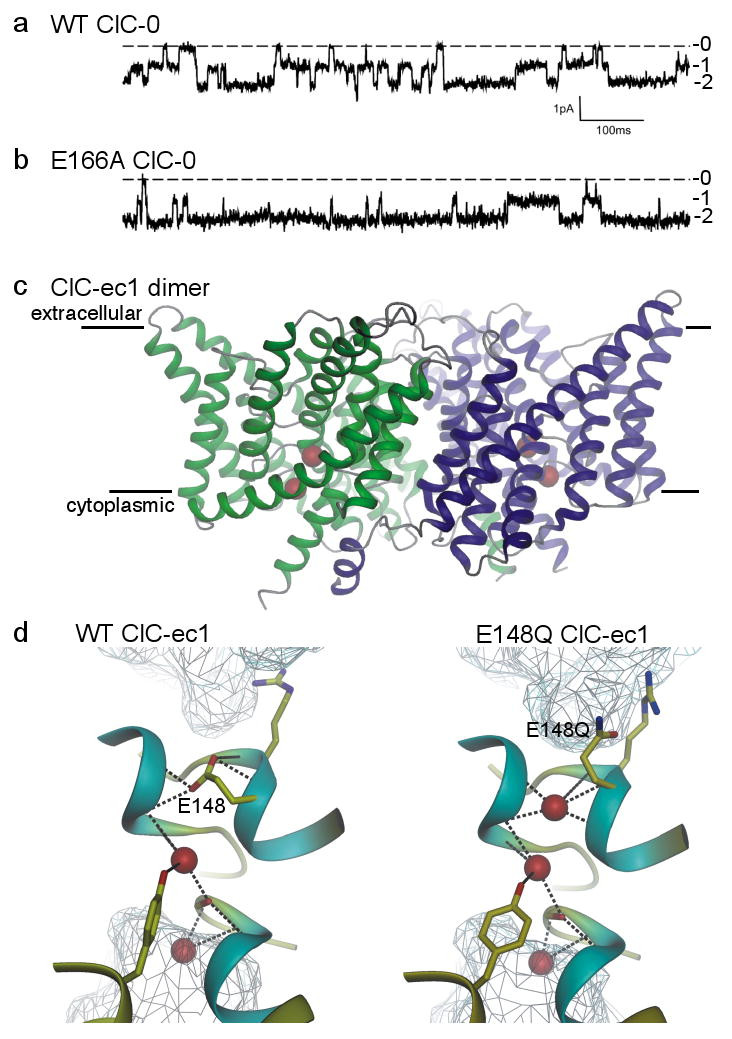{width=732 height=1039}